BIOL126 Lecture Notes - Lecture 5: Afferent Nerve Fiber, Schwann Cell, Axon Terminal
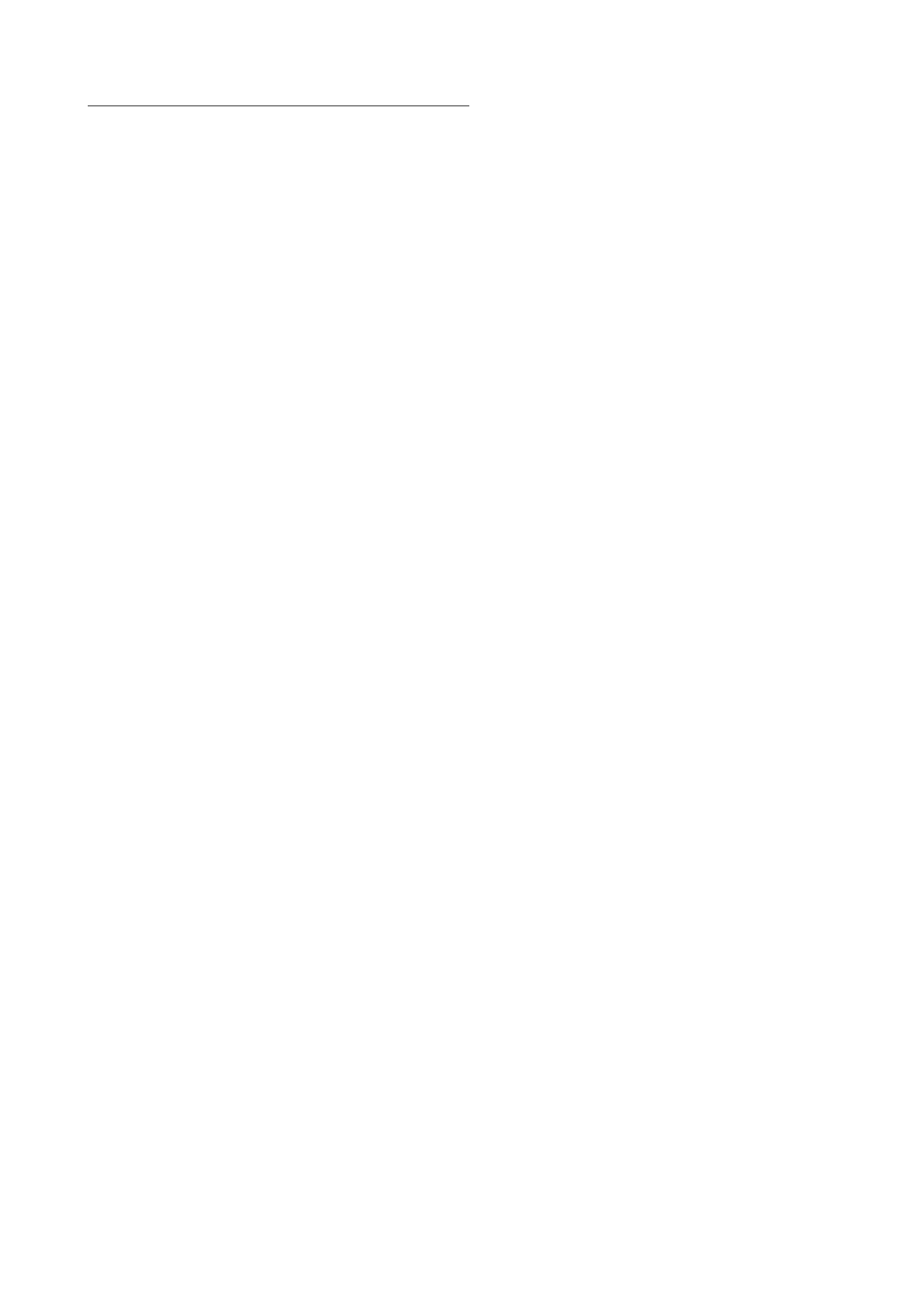
Overview Nervous System Structure and Divisions
3.1 Overview of structure, function and location
3.1.1 the functional divisions of the nervous system
3.1.1.1 sensory and motor
Sensory:
-afferent nerves
-brings sensory information to CNS from peripheral sensory receptors
Motor:
-efferent nerves
-takes commands from CNS to effectors
3.1.1.2 somatic and autonomic
Somantic- conscious control
Autonomic- involuntary/subconscious control
3.1.2 the differences between the central nervous system and the peripheral nervous
systems
CNS:
-comprising brain, brainstem and spinal cord, plus supporting cells and tissues
-Integrates, processes and co-ordinates both incoming sensory information and outgoing motor
commands
PNS:
-Delivers sensory information to CNS and carries motor commands to peripheral tissues/eclls
-comprising all neural tissue outside CNS including supporting cells and tissues
-Bundles of axons along with blood vessels and CT comprise nerves
3.2 The basic arrangement of the cells found in the central and peripheral nervous systems
including the following:
3.2.1 Neurons: including motor neurons, sensory neurons and interneurons;
Neuron- a cell in neural tissue that is specialised for intracellular communication through
1. changes in membrane potential, and
2. Synaptic conditions
Motor/ efferent neuron- carry instructions from CNS to peripheral effectors in a peripheral tissue,
organ or organ system
Sensory/ afferent neuron- deliver information from sensory receptors to CNS, located in a
peripheral sensory ganglia
Interneurons- located between sensory and motor neurone, distribute sensory information and
coordinate motor activity
3.2.2 Glial cells notably astrocytes, oligodendrocytes (CNS) and Schwann cells (PNS)
Supporting cells, glial cells, have functions essential to the survival and functionality of neurons
and to preserving the physical and biochemical structure of neural tissue, they seperate and
protect the neurons, provide a supportive framework for neural tissue, act as phagocytes and help
regulate the composition of the interstitial fluid
Types of glial cells:
Astrocytes- the largest and most numerous neuroglia
Oligodendrocytes- responsible for myelination of CNS axons
Schwann cells- ensheath axons in PNS , a singe cell may myelinate one segment of an axon or
enclose segments of several unmyelinated axons
3.3 The structure and function of a neuron
-Neuron is the basic functional unit; groups of neurons arranged with cll bodies together and
axons bundled together
-role to receive and transmit extremely fast electrochemical messages
-comprised of dendrites, cell body, axon, telodendria and axon terminals
3.4 What the ‘nucleus’ and ’ganglion’ are and what they do in the nervous system
Ganglia- collections of neural cell bodies outside the CNS in PNS (singular- ganglion)
find more resources at oneclass.com
find more resources at oneclass.com
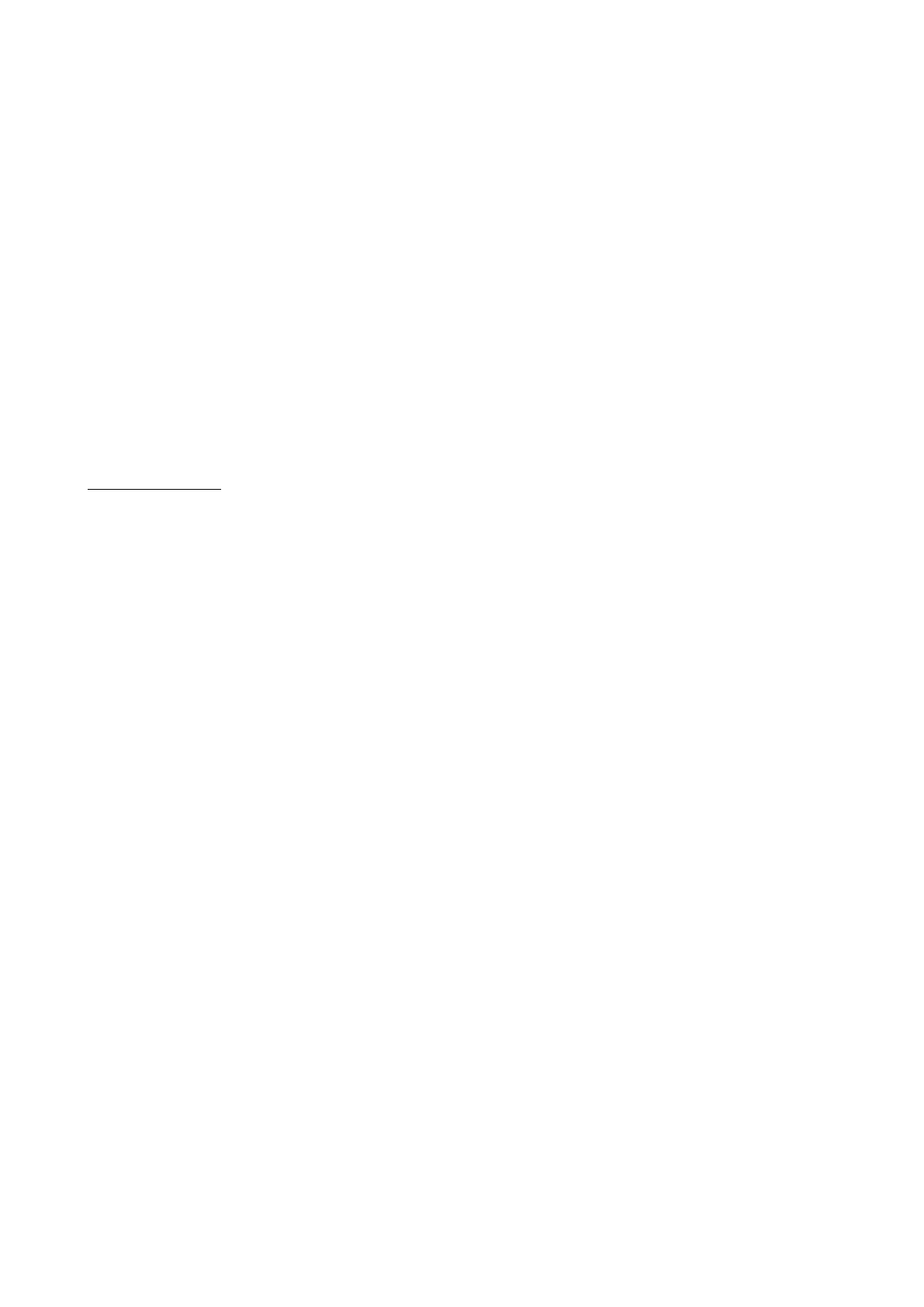
Nucleus- a cellular organelle that contains DNA, RNA, and proteins; in the CNS, a mass go grey
matter
3.5 The difference between grey matter and white matter
Grey matter- areas in the central nervous system that are dominated by neutron cell bodies,
neuroglia, and unmyelinated axons
White matter- regions in the central nervous system that are dominated by myelinated axons
3.6 Myelination
3.6.1 Where myelin is found in the central and peripheral nervous systems
-An insulating sheath around an axon; consists of multiple layers of neuroglia membrane;
significantly increases the nerve impulse propagation rate along the axon
-Extensions of the plasma membrane of Oligodendrocytes (in CNS) and Schwann cells (in PNS)
3.6.2 Where it is produced
-Schwann cells produce myelin
3.6.3 What myelin does and how this benefits the body
-Speeds the conduction of nerve impulses
-increased conduction speed increases NS information processing speed
Neural Messages
3.7 The nerve membrane; what the resting membrane potential is, and why it is needed
All living cells have a membrane potential that caries from moment to moment depending on the
activities of the cell. The resting membrane potential is the membrane potential of an unstimulated,
resting cell. All neural activities begin with a change in the resting membrane potential of a neuron.
normally between -10mV to -100mV, with the minus sign signifying that the inner surface of the
plasma membrane contains an excess of negative charges compared with the outside.
3.8 How nerve impulses are transmitted
3.8.1 the role of ion channels and the sodium-potassium pump
Intracellular concentration of potassium ions (K+) is relatively high, so these ions tend to move out
of the cell through potassium leak channels. Similarly, the extracellular concentration of sodium
ions (Na+) is relatively high, so sodium ions move into the cell through sodium leak channels. Both
of these movements are driven by a concentration gradient, or chemical gradient.
Sodium- potassium exchange pumps maintain the concentration of sodium and potassium ions
across the plasma membrane.
3.8.2 graded potentials
A typical stimulus produces a temporary, localised change in the resting membrane potential. The
effect, which decreases with distance from the stimulus, is called a graded potential.
3.9 Action potentials: how they are initiated and propagated
If the graded potential is large enough, it triggers an action potential in the membrane of the icon.
An action potential is an electrical impulse that is propagated (spread) along the surface of an axon
and does not diminish as it moves away from its source. This impulse travels along the axon to one
or more synapses.
An action potential (message) is relayed from one location to another in a series of steps. At each
step, the message is repeated. Action potentials may travel along an axon by continuous
propagation or by saltatory propagation.
3.9.1 what depolarisation, repolarisation and hyperpolarisation and refractory period
(relative and absolute) are
The plasma membrane does not respond normally to additional depolarising stimuli from the time
an action potential begins until the normal resting membrane potential has stabilised. This period is
known as the refractory portion go the membrane. The membrane cannot respond to further
simulation from the moment the voltage-gated sodium channels open at threshold until sodium
channels inactivation ends. This initial part is called absolute refractory. The relative refractory
find more resources at oneclass.com
find more resources at oneclass.com
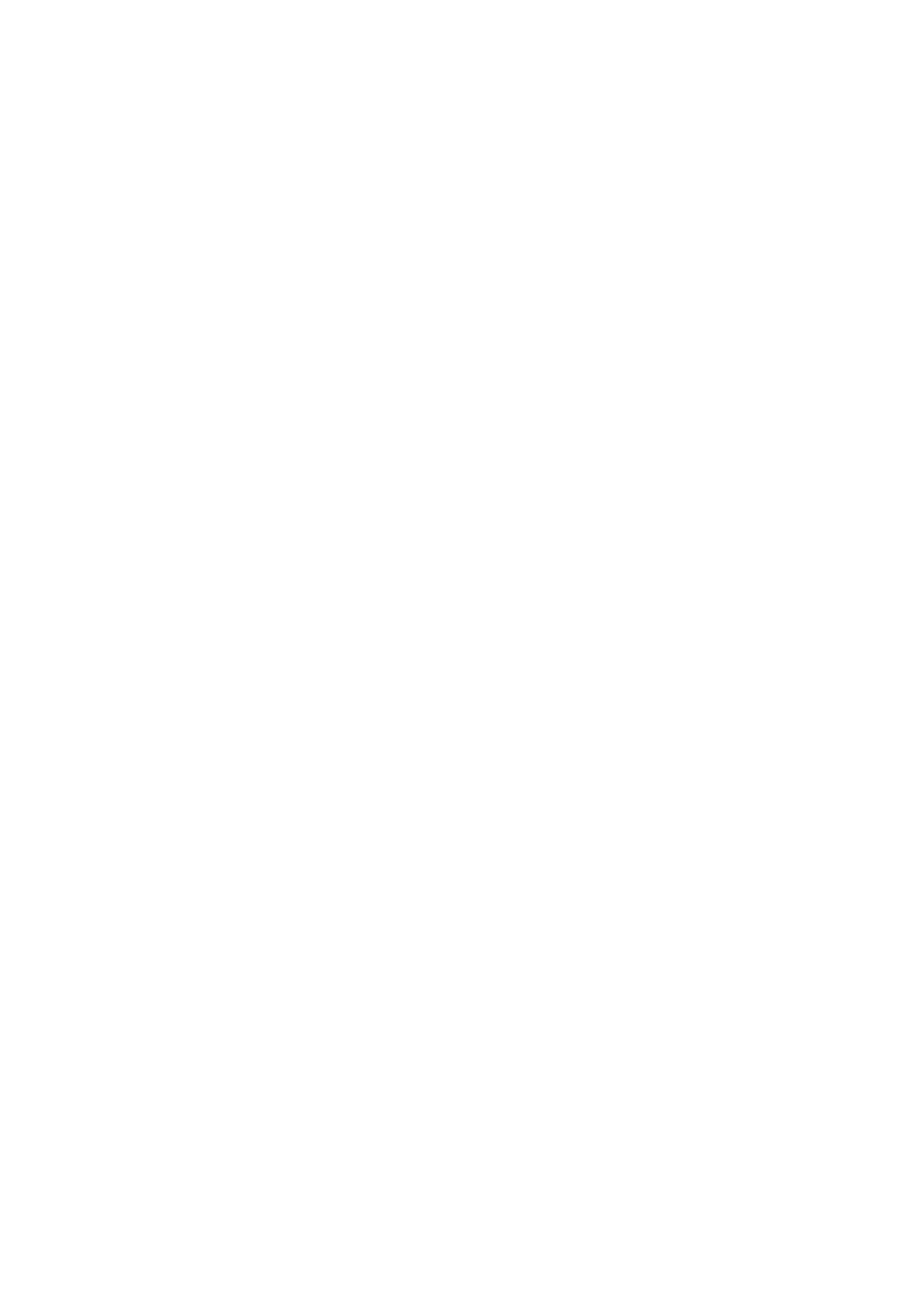
period begins when the sodium channels regain their normal resting condition, and continues until
the membrane potential stabilises at resting levels.
Any shift from the resting membrane potential toward a more positive potential is called a
depolarisation.
The process of restoring the normal resting membrane potential after depolarisation is called
repolarisation.
The loss of positive ions produces hyperpolarisation, an increase in the negatively of the resting
membrane potential.
3.10 Non-gated and gated ion channels;
3.10.1 their roles in maintaining the resting membrane potential and developing an
action potential
Passive channels, or leak channels, are always open. Their permeability can vary from moment to
moment as the proteins that make up the channel change shape in response to local conditions.
Leak channels are important in establishing the normal resting membrane potential of the cell.
Active channels, or gated channels, open or close in response to specific stimuli. Each gated
channel can be in one of three states; 1) closed but capable of opening, 2) open (activated) or, 3)
closed and incapable of opening (inactivated).
Chemically gated channels open or close when they bind specific chemicals. the receptors that
bind acetylcholine (ACh) at the neuromuscular junction are chemically gated channels. These are
most abundant on the dendrites and cell body of a neuron, the areas where most synaptic
communication occurs.
Voltage-gated channels open or close in response to changed in the membrane potential. They are
characteristic of areas of excitable membrane, a membrane capable of generating and propagating
an action potential. The most important are voltes-gated sodium, calcium and potassium channels.
These sodium channels have two gates that function independently; an activation gate that open
on stimulus, letting sodium ions into the cell, and an inactivation gate that closes to stop the entry
of sodium ions.
Mechanically gated channels open or close in response to physical distortion of the membrane
surface. Such channels are important in sensory receptors that respond to touch, pressure, or
vibration.
3.11 How the size of a nerve fibre, and whether or not it is myelinated relates to its and
conduction velocity
Nerve fibre diameter (not nerve diameter) determines nerve conduction velocity. Fibre diameter is
the diameter of the entire nerve fibre: axon + neurilemma + myelin sheath in myelinated axons and
axon + neurilemma in unmyelinated axons. The bulgy Schwann cell body will average out with the
rest of the myelin and the neurilemma is so thin it can be ignored. Thus, we can think:
unmyelinated fibre diameter = axon diameter and myelinated fibre diameter = (myelin+axon)
diameter. Nerve conduction velocity is determined by the speed of transmission of the action
potential along the nerve fibre.
3.12 Saltatory conduction, and how it occurs
Saltatory propagation in the CNS and PNS carries action potentials along an axon much more
rapidly than does continuous propagation. Continuous propagation can not occur along a
myelinated axon, because myelin increases resistance to the flow of ions across the membrane.
ions can readily cross the plasma membrane only at nodes. As a result, only the nodes with their
voltage-gated channels can respond to a depolarising stimulus.
When an action potential appears at the initial segment of a myelinated axon, the local Current
skips the internodes and depolarises the closest node to threshold. The action potential “jumps”
from node to node. In addition to being faster, saltatory propagation uses proportionately less
find more resources at oneclass.com
find more resources at oneclass.com
Document Summary
3. 1. 1 the functional divisions of the nervous system. Brings sensory information to cns from peripheral sensory receptors. 3. 1. 2 the differences between the central nervous system and the peripheral nervous systems. Comprising brain, brainstem and spinal cord, plus supporting cells and tissues. Integrates, processes and co-ordinates both incoming sensory information and outgoing motor commands. Delivers sensory information to cns and carries motor commands to peripheral tissues/eclls. Comprising all neural tissue outside cns including supporting cells and tissues. Bundles of axons along with blood vessels and ct comprise nerves. 3. 2 the basic arrangement of the cells found in the central and peripheral nervous systems including the following: 3. 2. 1 neurons: including motor neurons, sensory neurons and interneurons; Neuron- a cell in neural tissue that is specialised for intracellular communication through: changes in membrane potential, and, synaptic conditions. Motor/ efferent neuron- carry instructions from cns to peripheral effectors in a peripheral tissue, organ or organ system.