MBG 3060 Lecture Notes - Lecture 3: Mutation, Allele, Mutation Rate
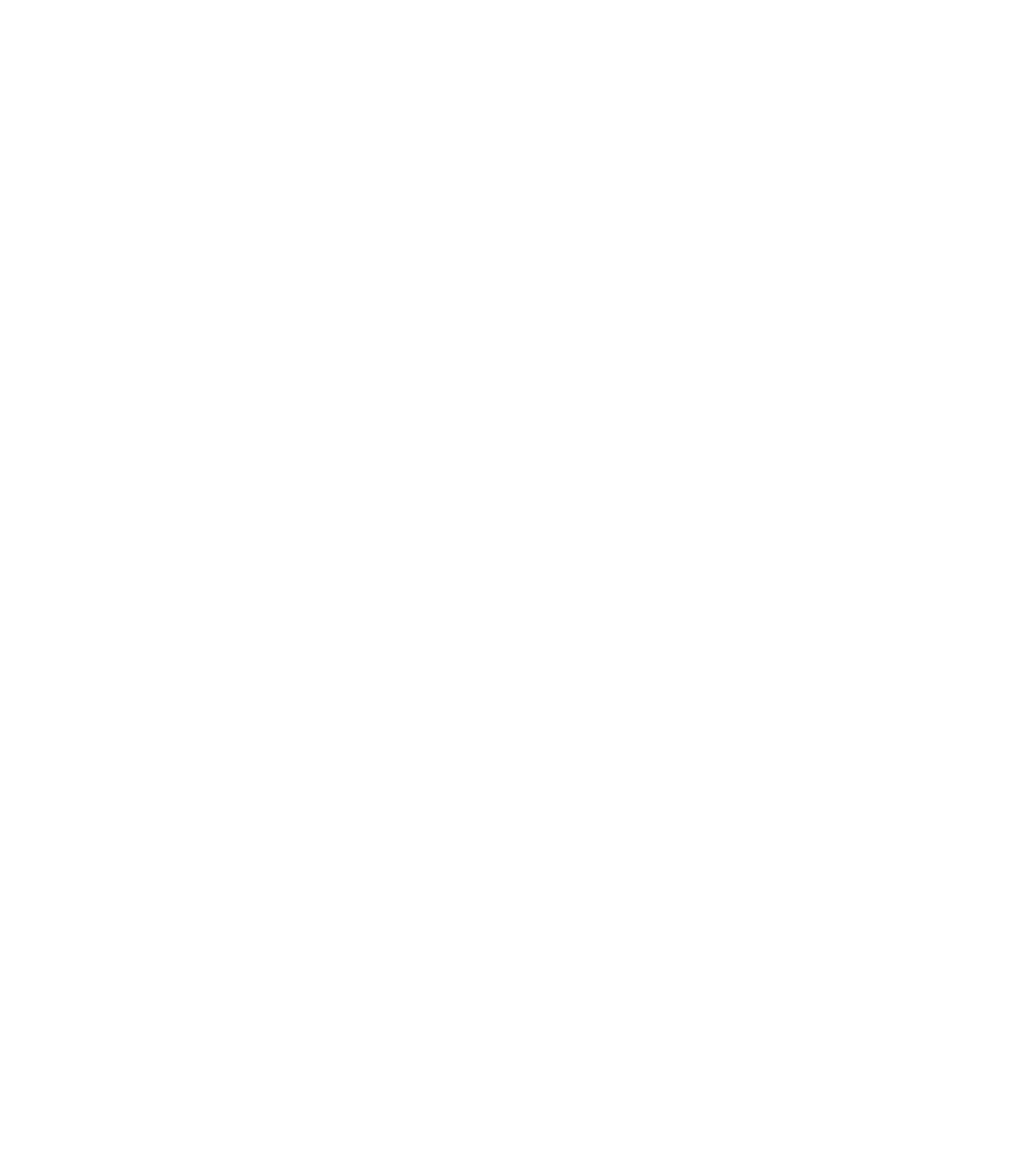
Migration -influx of alleles
•
Selection -favorable alleles have increasing frequency
•
Mutation -creation of new alleles
•
Three factors that mess up Hardy-Weinberg Equilibrium are migration,
selection and mutation which alter allele frequencies.
Movement of alleles from one population to the next
•
If allele frequencies are the same between two populations, migration
has no effect
•
Population 1: A=p1, a=q1
○
Population 2: A=p2, a=q2
○
*population 2 migrates into population 1
○
--> population 1 after migration: p1'= mp2 + (1-m)p1 = p1 +
m(p2-p1)
○
M is the migration rate = proportion of the total combined number
of individuals in the new population 1 that came from population
2
○
Example:
•
M=5%=0.05
○
P1' = m(p2-p1) + p1 = 0.05(0-1) + 1 = 0.95
○
Q1= 1-p1' = 1-0.95 = 0.05
○
Ex. Wild population of squirrels are all black [f(B)=1, f(b)=0]. A
researcher releases grey squirrels [f(B)=0, f(b)=1] that make up 5% of
the population. Assuming colour is controlled by a single locus (B or b),
what is the frequency of the grey allele?
•
M = migrants
!
1-m = natives
!
Starting population: generation 0
○
Pure migrants = m^2
!
Mixed = (1-m)m
!
Pure natives = (1-m)^2
!
Population after one year of random mating:
○
Tracking proportion of individuals in combined population by origin:
•
M = (p1' -p1) / (p2-p1)
○
Calculating migration rate:
•
1p1= p1'
○
1p1 = 0p1 (1-m) + mp2
○
kp1 = k-1p1 (1-m) + mp2
○
If these are not constant calculate each generation separately
!
*m is assumed to be constant and the size of the native population
is assumed constant as a proportion
○
2p1 = 0p1 (1-m)(1-m) + [1-(1-m)(1-m)]p2
!
Note: p1 is decreased by a proportion of (1-m) in each generation
○
kp1 = 0p1 (1-m)^k + [1-(1-m)^k]p2
!
So, after k generations,
○
(1-m)^k = (kp1 - p2) / (0p1- p2)
!
Reordering, we get…
○
Continued migration on population allele frequency:
•
U=migration rate for males
!
V = migration rate for females
!
Pm2 = frequency for males in population 2
!
Pf2 = frequency for females in population 2
!
P1= 1/2 {[upm2 + (1-u)p1] + [vpf2 + (1-v)p1]}
○
P1= 1/2 {[20/70(1.0) + (1-(20/70))(.04)] + [0(1.0) + (1-0)
(0.04)]} = 0.177
!
*if not given number of M and F assume 50:50 ratio
!
Ex. P1=0.04 with a population of 50M and 50F--> bring in 20
males with p2 =1.0
○
Differential Migration by Gender
•
Migration
The degree to which a population responds to these environmental
pressures is usually proportional to the severity of the pressure
○
These rapid changes may put the population through a
"bottleneck" -event where only the fittest few survive
!
A gradual change (e.g. climate change) lets a population gradually
adapt and would be less likely to cause rapid changes in allele
frequencies compared to events like prolonged drought or an ice
age
○
The extreme example of natural selection is extinction where they
cannot adapt quickly enough
○
Natural Selection -process that changes allele frequencies in a wild
population
•
Notion of selection being intentional and driven by a human
decision making process
○
Artificial Selection -based on human intervention
•
A1A1 A1A2 A2A2
Genotypic frequency in
parents
P^2 2pq Q^2
Fitness of Genotypes wW(1-s1) W(1-s2)
*usually w=
1
(1-s1) (1-s2)
Genotypic frequency after
selection
P^2 2(1-s1)pq (1-s2)q^2
*s1 and s2 = selection proportion or the individuals eliminated
from the population
Fitness and Allele Frequency:
•
A1A1 A1A2 A2A2
Frequency P^2 2pq Q^2
Number 810 180 10
Fitness 1 1 0.1
After Selection 810 180 1
P' = 810/991 + 1/2(180/991) = 0.908
Change in P = 0.908-0.9 = 0.008
Example: starting…p=f(A1)=0.9, q=f(A2)=0.1
•
P1 = [p0 (1-s1q0)] / [1-2*s1p0q0 -s2q0^2]
○
Total survivors = p0^2 + 2(1-s1)p0q0 + (1-s2)q0^2
○
P0=0.6, q0=0.4, s1=0.25, s2=0.50
!
P1 = 0.675 *using first equation above
!
Example:
○
Selection: One Preferred Genotype
•
AA: fitness=1
○
Aa: fitness = 1
○
'aa': fitness = 1-s
○
NOTE: if s=1, 'a' is a lethal recessive allele
○
Total survivors (aa) = 1 -q0^2
○
P1= p0/ (1-s*q0^2)
○
Selection Against 'aa'
•
AA Aa aa
Generation 0 P0^2 2pq Q0^2
Fitness 1 1 0
New Frequency P0^2 2p0q0 0
New Proportion P0^2/T 2P0q0 /T 0
AA Aa aa
Generation 1 P1^2 2pq Q1^2
Expressed as
f(q0)
1/ (1+q0)^2 2q0 / (1+q0)^2 Q0^2 /(1+q0)^2
Fitness 1 1 0
--> pn= [1 + (n-1)q0] / [1 + nq0]
Qn= q0/ (1+ nq0)
!
Pn= (1+ (n-1)q0)/ (1+ nq0)
!
When the aa genotype is lethal, the frequencies of the A and a
alleles in the n(th) generation as a function of the initial
frequencies are:
○
N = (1/qn) -(1/q0)
!
*n should always be rounded up
!
How to calculate 'n':
○
Selection over time -aa Lethal
•
AA Aa aa
Frequency 0.36 0.48 0.16
Fitness 10.75 0.5
F(A) = 0.36 + 0.75(0.48/2) = 0.54, F(a) = 0.75(0.48/2) +
0.5(0.16) = 0.26
Total = 0.54 + 0.26 = 0.8
P1= 0.54/0.8 = 0.675 , q1 = 0.26/0.8 = 0.325
Therefore, change in p = 0.675 -0.6 = 0.075
Ex. Let p=0.6, q=0.4 and s=0.5
○
Selection with Co-Dominance
•
If we select for Aa individuals, we won’t loose any alleles from
the population but if repeated over generations, we will eventually
reach equilibrium
○
AA Aa aa
Frequency P0^2 2pq Q0^2
Fitness 1-s1 1 1-s2
Selection for Heterozygotes
•
Selection
Selection Summary:
Gene Action Fitness Frequency of A Allele
Dominance, selection for
A_
AA: 1
Aa: 1
'aa': 1-s
P1= p0/ (1 -s*q0^2)
*pE--> fixed
Dominance, selection for
aa
AA: 1-s
Aa: 1-s
'aa': 1
P1= p0(1-s) / (1 -s*p0(1+q0))
*pE--> 0, A is lost
Codominance AA: 1
Aa: 1-
0.5s
'aa': 1-s
P1= p0(1-0.5sq0) / (1 -s*q0)
*pE--> fixed
Incomplete Dominance AA: 1
Aa: 1-s1
'aa': 1-s2
P1= p0(1-s1q0) / (1 -2s1*p0q0- s2q0^
2)
*pE--> fixed
Over-Dominance AA: 1-s1
Aa: 1
'aa': 1-s2
P1= p0(1-s1p0) / (1 -2s1*p0^2 - s2q0^
2)
*pE= s2/ (s2+ s1)
A --> a at a rate 'u'
○
'a'---> A at a rate 'v'
○
Change in P = v(1-p) -up
○
Change in Q = u(1-q) -vq
○
pE = v/(u+v)
!
qE = u/(u+v)
!
At equilibrium, change of p = change of q = 0
○
Defining mutation rates:
•
At equilibrium amount of mutation = selection (codominance)
○
Change in q = u(1-q) -vq = [sq^2 (1-q)]/ (1-sq^2)
○
-vq is "really small" -relatively unimportant
!
Sq^2 is inconsequential compared to 1 and simplifying
!
If q is small:
○
--> u(1-q) = sq^2 (1-q)
○
Therefore, q = sq.rt(u/s)
○
Mutation vs. Selection
•
Given mutation rate of A1-->A2 = 0.0001 with 's' against A2 = 0.1
○
Q = sq. rt. (0.0001/0.1) = 0.001 0.0316
○
Example:
•
Albino (aa): f(a)=qE=0.05, s=1
○
qE = 0.05 = sq. rt( u/1)
○
Therefore, u = 0.0025
○
Example:
•
Change of p = sq *if p is close to 1
○
If selection balances mutation, up=sq
!
But, when p is close to 1, up=u
!
So, u=sq and qE= u/s
!
Mutation rate u (A1-->A2) causes the change of p of u*p which
must equal the gain of change in p due to selection
○
With Incomplete Dominance:
•
Mutation
Let s1=0, s2=0.9
T = total individuals remaining
Then,
P1 = 1-q1 = 1 -(q0 / (1+q0)) = 1/ (1+q0)
= (1/2*.5*.6*.4) / (1 -.5*.4)
= 0.075
Change in P = (1/2*s*p0*q0)/(1 -s*q0)
Change in P = [pq(s2q -s1p)] / [1-(s1P^2) -(s2q^2)]
*if we reach equilibrium, change in p = 0
SO, pE = s2/(s1+s2) and qE = s1/(s2+s1)
3. Migration, Selection & Mutation
Saturday,*February*4,*2017
1:36*PM
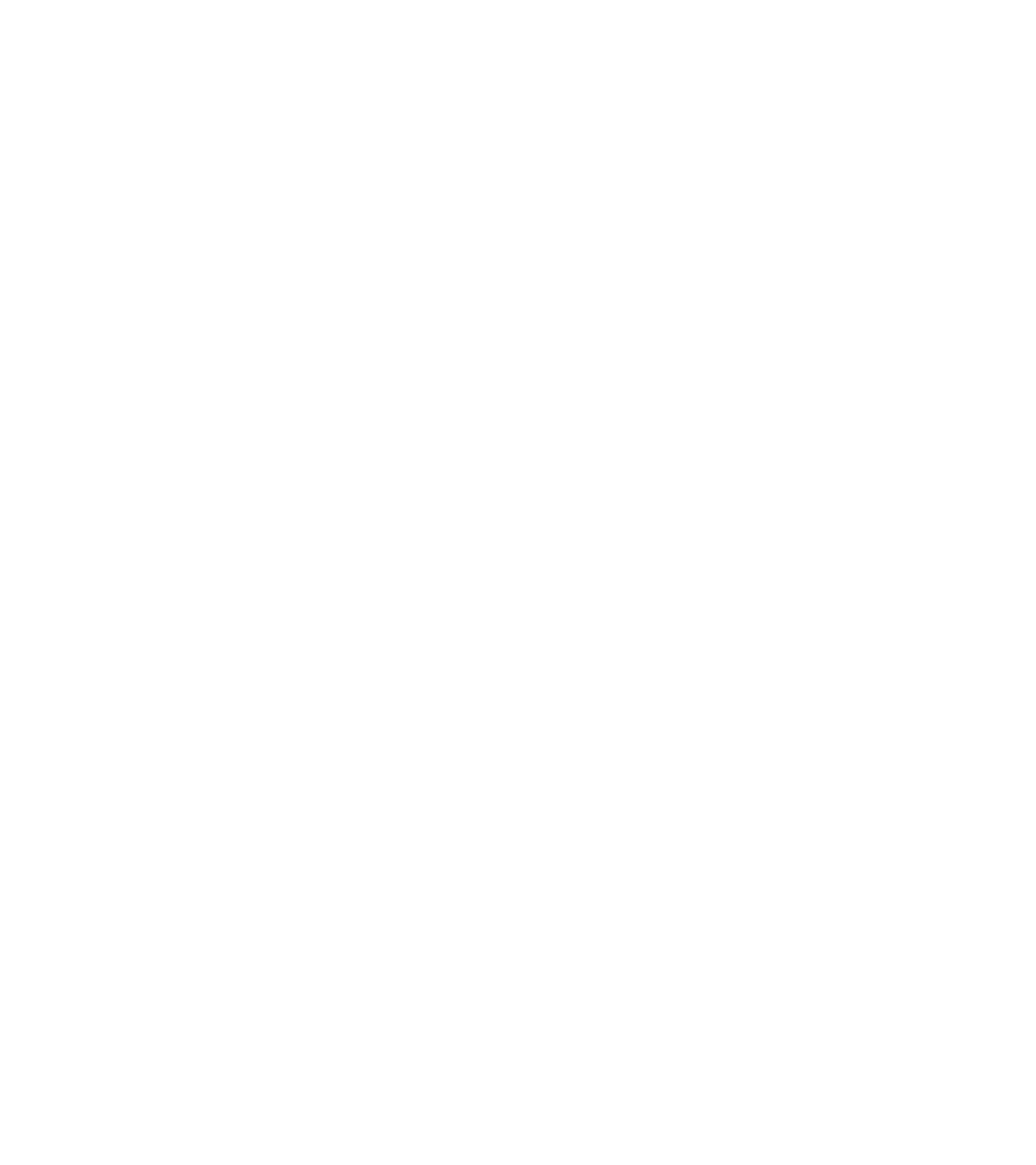
Migration -influx of alleles
•
Selection -favorable alleles have increasing frequency
•
Mutation -creation of new alleles
•
Three factors that mess up Hardy-Weinberg Equilibrium are migration,
selection and mutation which alter allele frequencies.
Movement of alleles from one population to the next
•
If allele frequencies are the same between two populations, migration
has no effect
•
Population 1: A=p1, a=q1
○
Population 2: A=p2, a=q2
○
*population 2 migrates into population 1
○
--> population 1 after migration: p1'= mp2 + (1-m)p1 = p1 +
m(p2-p1)
○
M is the migration rate = proportion of the total combined number
of individuals in the new population 1 that came from population
2
○
Example:
•
M=5%=0.05
○
P1' = m(p2-p1) + p1 = 0.05(0-1) + 1 = 0.95
○
Q1= 1-p1' = 1-0.95 = 0.05
○
Ex. Wild population of squirrels are all black [f(B)=1, f(b)=0]. A
researcher releases grey squirrels [f(B)=0, f(b)=1] that make up 5% of
the population. Assuming colour is controlled by a single locus (B or b),
what is the frequency of the grey allele?
•
M = migrants
!
1-m = natives
!
Starting population: generation 0
○
Pure migrants = m^2
!
Mixed = (1-m)m
!
Pure natives = (1-m)^2
!
Population after one year of random mating:
○
Tracking proportion of individuals in combined population by origin:
•
M = (p1' -p1) / (p2-p1)
○
Calculating migration rate:
•
1p1= p1'
○
1p1 = 0p1 (1-m) + mp2
○
kp1 = k-1p1 (1-m) + mp2
○
If these are not constant calculate each generation separately
!
*m is assumed to be constant and the size of the native population
is assumed constant as a proportion
○
2p1 = 0p1 (1-m)(1-m) + [1-(1-m)(1-m)]p2
!
Note: p1 is decreased by a proportion of (1-m) in each generation
○
kp1 = 0p1 (1-m)^k + [1-(1-m)^k]p2
!
So, after k generations,
○
(1-m)^k = (kp1 - p2) / (0p1- p2)
!
Reordering, we get…
○
Continued migration on population allele frequency:
•
U=migration rate for males
!
V = migration rate for females
!
Pm2 = frequency for males in population 2
!
Pf2 = frequency for females in population 2
!
P1= 1/2 {[upm2 + (1-u)p1] + [vpf2 + (1-v)p1]}
○
P1= 1/2 {[20/70(1.0) + (1-(20/70))(.04)] + [0(1.0) + (1-0)
(0.04)]} = 0.177
!
*if not given number of M and F assume 50:50 ratio
!
Ex. P1=0.04 with a population of 50M and 50F--> bring in 20
males with p2 =1.0
○
Differential Migration by Gender
•
Migration
The degree to which a population responds to these environmental
pressures is usually proportional to the severity of the pressure
○
These rapid changes may put the population through a
"bottleneck" -event where only the fittest few survive
!
A gradual change (e.g. climate change) lets a population gradually
adapt and would be less likely to cause rapid changes in allele
frequencies compared to events like prolonged drought or an ice
age
○
The extreme example of natural selection is extinction where they
cannot adapt quickly enough
○
Natural Selection -process that changes allele frequencies in a wild
population
•
Notion of selection being intentional and driven by a human
decision making process
○
Artificial Selection -based on human intervention
•
A1A1 A1A2 A2A2
Genotypic frequency in
parents
P^2 2pq Q^2
Fitness of Genotypes wW(1-s1) W(1-s2)
*usually w=
1
(1-s1) (1-s2)
Genotypic frequency after
selection
P^2 2(1-s1)pq (1-s2)q^2
*s1 and s2 = selection proportion or the individuals eliminated
from the population
Fitness and Allele Frequency:
•
A1A1 A1A2 A2A2
Frequency P^2 2pq Q^2
Number 810 180 10
Fitness 1 1 0.1
After Selection 810 180 1
P' = 810/991 + 1/2(180/991) = 0.908
Change in P = 0.908-0.9 = 0.008
Example: starting…p=f(A1)=0.9, q=f(A2)=0.1
•
P1 = [p0 (1-s1q0)] / [1-2*s1p0q0 -s2q0^2]
○
Total survivors = p0^2 + 2(1-s1)p0q0 + (1-s2)q0^2
○
P0=0.6, q0=0.4, s1=0.25, s2=0.50
!
P1 = 0.675 *using first equation above
!
Example:
○
Selection: One Preferred Genotype
•
AA: fitness=1
○
Aa: fitness = 1
○
'aa': fitness = 1-s
○
NOTE: if s=1, 'a' is a lethal recessive allele
○
Total survivors (aa) = 1 -q0^2
○
P1= p0/ (1-s*q0^2)
○
Selection Against 'aa'
•
AA Aa aa
Generation 0 P0^2 2pq Q0^2
Fitness 1 1 0
New Frequency P0^2 2p0q0 0
New Proportion P0^2/T 2P0q0 /T 0
AA Aa aa
Generation 1 P1^2 2pq Q1^2
Expressed as f(q0) 1/ (1+q0)^2 2q0 / (1+q0)^
2
Q0^2 /(1+q0)^
2
Fitness 1 1 0
--> pn= [1 + (n-1)q0] / [1 + nq0]
Qn= q0/ (1+ nq0)
!
Pn= (1+ (n-1)q0)/ (1+ nq0)
!
When the aa genotype is lethal, the frequencies of the A and a
alleles in the n(th) generation as a function of the initial
frequencies are:
○
N = (1/qn) -(1/q0)
!
*n should always be rounded up
!
How to calculate 'n':
○
Selection over time -aa Lethal
•
AA Aa aa
Frequency 0.36 0.48 0.16
Fitness 10.75 0.5
F(A) = 0.36 + 0.75(0.48/2) = 0.54, F(a) = 0.75(0.48/2) +
0.5(0.16) = 0.26
Total = 0.54 + 0.26 = 0.8
P1= 0.54/0.8 = 0.675 , q1 = 0.26/0.8 = 0.325
Therefore, change in p = 0.675 -0.6 = 0.075
Ex. Let p=0.6, q=0.4 and s=0.5
○
Selection with Co-Dominance
•
If we select for Aa individuals, we won’t loose any alleles from
the population but if repeated over generations, we will eventually
reach equilibrium
○
AA Aa aa
Frequency P0^2 2pq Q0^2
Fitness 1-s1 1 1-s2
Selection for Heterozygotes
•
Selection
Selection Summary:
Gene Action Fitness Frequency of A Allele
Dominance, selection for
A_
AA: 1
Aa: 1
'aa': 1-s
P1= p0/ (1 -s*q0^2)
*pE--> fixed
Dominance, selection for
aa
AA: 1-s
Aa: 1-s
'aa': 1
P1= p0(1-s) / (1 -s*p0(1+q0))
*pE--> 0, A is lost
Codominance AA: 1
Aa: 1-0.5s
'aa': 1-s
P1= p0(1-0.5sq0) / (1 -s*q0)
*pE--> fixed
Incomplete Dominance AA: 1
Aa: 1-s1
'aa': 1-s2
P1= p0(1-s1q0) / (1 -2s1*p0q0- s2q0^
2)
*pE--> fixed
Over-Dominance AA: 1-s1
Aa: 1
'aa': 1-s2
P1= p0(1-s1p0) / (1 -2s1*p0^2 - s2q0^
2)
*pE= s2/ (s2+ s1)
A --> a at a rate 'u'
○
'a'---> A at a rate 'v'
○
Change in P = v(1-p) -up
○
Change in Q = u(1-q) -vq
○
pE = v/(u+v)
!
qE = u/(u+v)
!
At equilibrium, change of p = change of q = 0
○
Defining mutation rates:
•
At equilibrium amount of mutation = selection (codominance)
○
Change in q = u(1-q) -vq = [sq^2 (1-q)]/ (1-sq^2)
○
-vq is "really small" -relatively unimportant
!
Sq^2 is inconsequential compared to 1 and simplifying
!
If q is small:
○
--> u(1-q) = sq^2 (1-q)
○
Therefore, q = sq.rt(u/s)
○
Mutation vs. Selection
•
Given mutation rate of A1-->A2 = 0.0001 with 's' against A2 = 0.1
○
Q = sq. rt. (0.0001/0.1) = 0.001 0.0316
○
Example:
•
Albino (aa): f(a)=qE=0.05, s=1
○
qE = 0.05 = sq. rt( u/1)
○
Therefore, u = 0.0025
○
Example:
•
Change of p = sq *if p is close to 1
○
If selection balances mutation, up=sq
!
But, when p is close to 1, up=u
!
So, u=sq and qE= u/s
!
Mutation rate u (A1-->A2) causes the change of p of u*p which
must equal the gain of change in p due to selection
○
With Incomplete Dominance:
•
Mutation
Let s1=0, s2=0.9
T = total individuals remaining
Then,
P1 = 1-q1 = 1 -(q0 / (1+q0)) = 1/ (1+q0)
= (1/2*.5*.6*.4) / (1 -.5*.4)
= 0.075
Change in P = (1/2*s*p0*q0)/(1 -s*q0)
Change in P = [pq(s2q -s1p)] / [1-(s1P^2) -(s2q^2)]
*if we reach equilibrium, change in p = 0
SO, pE = s2/(s1+s2) and qE = s1/(s2+s1)
3. Migration, Selection & Mutation
Saturday,*February*4,*2017 1:36*PM
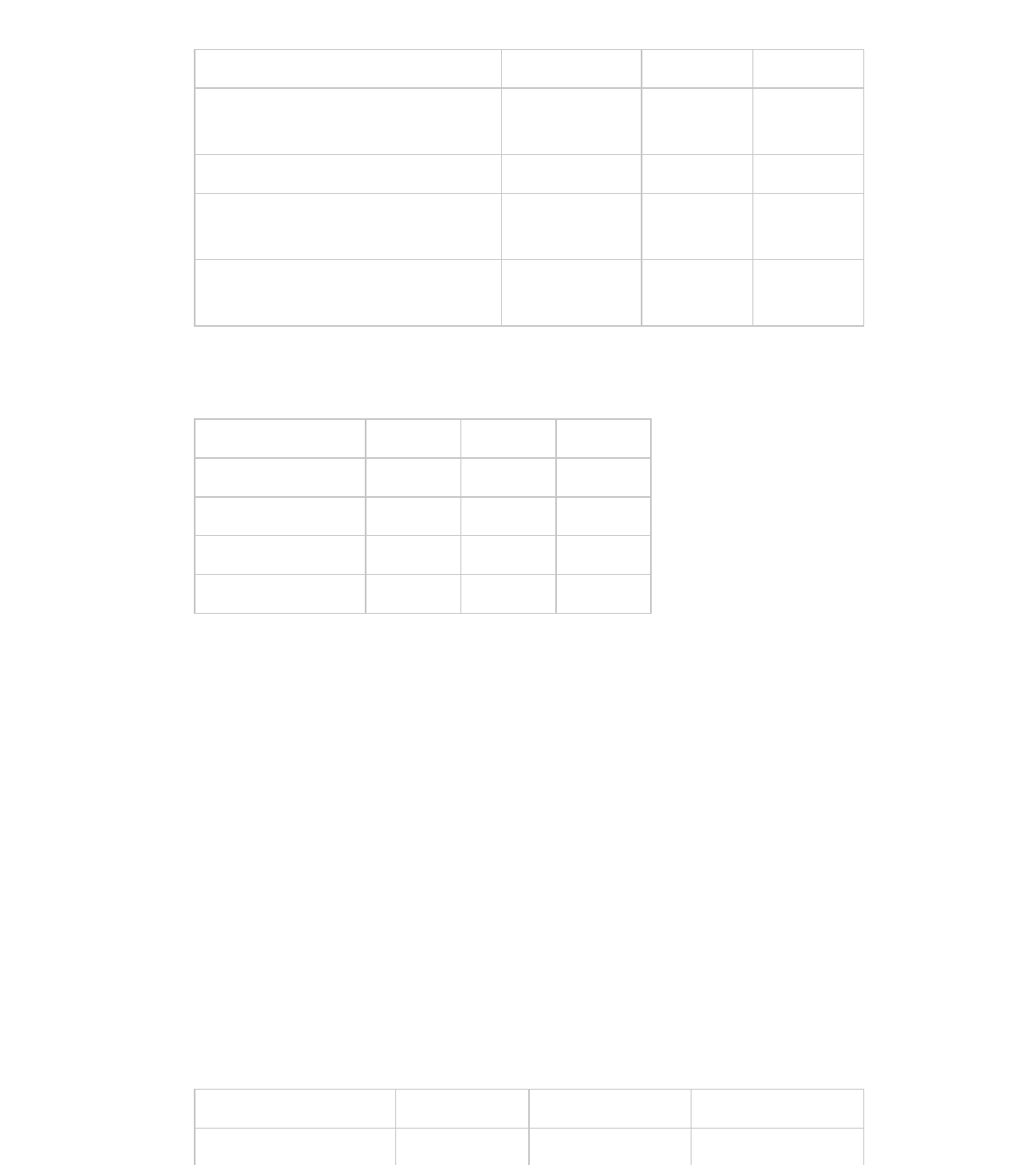
Migration -influx of alleles
•
Selection -favorable alleles have increasing frequency
•
Mutation -creation of new alleles
•
Three factors that mess up Hardy-Weinberg Equilibrium are migration,
selection and mutation which alter allele frequencies.
Movement of alleles from one population to the next
•
If allele frequencies are the same between two populations, migration
has no effect
•
Population 1: A=p1, a=q1
○
Population 2: A=p2, a=q2
○
*population 2 migrates into population 1
○
--> population 1 after migration: p1'= mp2 + (1-m)p1 = p1 +
m(p2-p1)
○
M is the migration rate = proportion of the total combined number
of individuals in the new population 1 that came from population
2
○
Example:
•
M=5%=0.05
○
P1' = m(p2-p1) + p1 = 0.05(0-1) + 1 = 0.95
○
Q1= 1-p1' = 1-0.95 = 0.05
○
Ex. Wild population of squirrels are all black [f(B)=1, f(b)=0]. A
researcher releases grey squirrels [f(B)=0, f(b)=1] that make up 5% of
the population. Assuming colour is controlled by a single locus (B or b),
what is the frequency of the grey allele?
•
M = migrants
!
1-m = natives
!
Starting population: generation 0
○
Pure migrants = m^2
!
Mixed = (1-m)m
!
Pure natives = (1-m)^2
!
Population after one year of random mating:
○
Tracking proportion of individuals in combined population by origin:
•
M = (p1' -p1) / (p2-p1)
○
Calculating migration rate:
•
1p1= p1'
○
1p1 = 0p1 (1-m) + mp2
○
kp1 = k-1p1 (1-m) + mp2
○
If these are not constant calculate each generation separately
!
*m is assumed to be constant and the size of the native population
is assumed constant as a proportion
○
2p1 = 0p1 (1-m)(1-m) + [1-(1-m)(1-m)]p2
!
Note: p1 is decreased by a proportion of (1-m) in each generation
○
kp1 = 0p1 (1-m)^k + [1-(1-m)^k]p2
!
So, after k generations,
○
(1-m)^k = (kp1 - p2) / (0p1- p2)
!
Reordering, we get…
○
Continued migration on population allele frequency:
•
U=migration rate for males
!
V = migration rate for females
!
Pm2 = frequency for males in population 2
!
Pf2 = frequency for females in population 2
!
P1= 1/2 {[upm2 + (1-u)p1] + [vpf2 + (1-v)p1]}
○
P1= 1/2 {[20/70(1.0) + (1-(20/70))(.04)] + [0(1.0) + (1-0)
(0.04)]} = 0.177
!
*if not given number of M and F assume 50:50 ratio
!
Ex. P1=0.04 with a population of 50M and 50F--> bring in 20
males with p2 =1.0
○
Differential Migration by Gender
•
Migration
The degree to which a population responds to these environmental
pressures is usually proportional to the severity of the pressure
○
These rapid changes may put the population through a
"bottleneck" -event where only the fittest few survive
!
A gradual change (e.g. climate change) lets a population gradually
adapt and would be less likely to cause rapid changes in allele
frequencies compared to events like prolonged drought or an ice
age
○
The extreme example of natural selection is extinction where they
cannot adapt quickly enough
○
Natural Selection -process that changes allele frequencies in a wild
population
•
Notion of selection being intentional and driven by a human
decision making process
○
Artificial Selection -based on human intervention
•
A1A1
A1A2
A2A2
Genotypic frequency in
parents
P^2
2pq
Q^2
Fitness of Genotypes
w
W(1-s1)
W(1-s2)
*usually w=
1
(1-s1)
(1-s2)
Genotypic frequency after
selection
P^2
2(1-s1)pq
(1-s2)q^2
*s1 and s2 = selection proportion or the individuals eliminated
from the population
Fitness and Allele Frequency:
•
A1A1
A1A2
A2A2
Frequency
P^2
2pq
Q^2
Number
810
180
10
Fitness
1
1
0.1
After Selection
810
180
1
P' = 810/991 + 1/2(180/991) = 0.908
Change in P = 0.908-0.9 = 0.008
Example: starting…p=f(A1)=0.9, q=f(A2)=0.1
•
P1 = [p0 (1-s1q0)] / [1-2*s1p0q0 -s2q0^2]
○
Total survivors = p0^2 + 2(1-s1)p0q0 + (1-s2)q0^2
○
P0=0.6, q0=0.4, s1=0.25, s2=0.50
!
P1 = 0.675 *using first equation above
!
Example:
○
Selection: One Preferred Genotype
•
AA: fitness=1
○
Aa: fitness = 1
○
'aa': fitness = 1-s
○
NOTE: if s=1, 'a' is a lethal recessive allele
○
Total survivors (aa) = 1 -q0^2
○
P1= p0/ (1-s*q0^2)
○
Selection Against 'aa'
•
AA
Aa
aa
Generation 0
P0^2
2pq
Q0^2
Fitness 1 1 0
New Frequency P0^2 2p0q0 0
New Proportion P0^2/T 2P0q0 /T 0
AA Aa aa
Generation 1 P1^2 2pq Q1^2
Expressed as f(q0) 1/ (1+q0)^2 2q0 / (1+q0)^2 Q0^2 /(1+q0)^
2
Fitness 1 1 0
--> pn= [1 + (n-1)q0] / [1 + nq0]
Qn= q0/ (1+ nq0)
!
Pn= (1+ (n-1)q0)/ (1+ nq0)
!
When the aa genotype is lethal, the frequencies of the A and a
alleles in the n(th) generation as a function of the initial
frequencies are:
○
N = (1/qn) -(1/q0)
!
*n should always be rounded up
!
How to calculate 'n':
○
Selection over time -aa Lethal
•
AA Aa aa
Frequency 0.36 0.48 0.16
Fitness 10.75 0.5
F(A) = 0.36 + 0.75(0.48/2) = 0.54, F(a) = 0.75(0.48/2) +
0.5(0.16) = 0.26
Total = 0.54 + 0.26 = 0.8
P1= 0.54/0.8 = 0.675 , q1 = 0.26/0.8 = 0.325
Therefore, change in p = 0.675 -0.6 = 0.075
Ex. Let p=0.6, q=0.4 and s=0.5
○
Selection with Co-Dominance
•
If we select for Aa individuals, we won’t loose any alleles from
the population but if repeated over generations, we will eventually
reach equilibrium
○
AA Aa aa
Frequency P0^2 2pq Q0^2
Fitness 1-s1 1 1-s2
Selection for Heterozygotes
•
Selection
Selection Summary:
Gene Action Fitness Frequency of A Allele
Dominance, selection for
A_
AA: 1
Aa: 1
'aa': 1-s
P1= p0/ (1 -s*q0^2)
*pE--> fixed
Dominance, selection for
aa
AA: 1-s
Aa: 1-s
'aa': 1
P1= p0(1-s) / (1 -s*p0(1+q0))
*pE--> 0, A is lost
Codominance AA: 1
Aa: 1-0.5s
'aa': 1-s
P1= p0(1-0.5sq0) / (1 -s*q0)
*pE--> fixed
Incomplete Dominance AA: 1
Aa: 1-s1
'aa': 1-s2
P1= p0(1-s1q0) / (1 -2s1*p0q0- s2q0^
2)
*pE--> fixed
Over-Dominance AA: 1-s1
Aa: 1
'aa': 1-s2
P1= p0(1-s1p0) / (1 -2s1*p0^2 - s2q0
^2)
*pE= s2/ (s2+ s1)
A --> a at a rate 'u'
○
'a'---> A at a rate 'v'
○
Change in P = v(1-p) -up
○
Change in Q = u(1-q) -vq
○
pE = v/(u+v)
!
qE = u/(u+v)
!
At equilibrium, change of p = change of q = 0
○
Defining mutation rates:
•
At equilibrium amount of mutation = selection (codominance)
○
Change in q = u(1-q) -vq = [sq^2 (1-q)]/ (1-sq^2)
○
-vq is "really small" -relatively unimportant
!
Sq^2 is inconsequential compared to 1 and simplifying
!
If q is small:
○
--> u(1-q) = sq^2 (1-q)
○
Therefore, q = sq.rt(u/s)
○
Mutation vs. Selection
•
Given mutation rate of A1-->A2 = 0.0001 with 's' against A2 = 0.1
○
Q = sq. rt. (0.0001/0.1) = 0.001 0.0316
○
Example:
•
Albino (aa): f(a)=qE=0.05, s=1
○
qE = 0.05 = sq. rt( u/1)
○
Therefore, u = 0.0025
○
Example:
•
Change of p = sq *if p is close to 1
○
If selection balances mutation, up=sq
!
But, when p is close to 1, up=u
!
So, u=sq and qE= u/s
!
Mutation rate u (A1-->A2) causes the change of p of u*p which
must equal the gain of change in p due to selection
○
With Incomplete Dominance:
•
Mutation
Let s1=0, s2=0.9
T = total individuals remaining
Then,
P1 = 1-q1 = 1 -(q0 / (1+q0)) = 1/ (1+q0)
= (1/2*.5*.6*.4) / (1 -.5*.4)
= 0.075
Change in P = (1/2*s*p0*q0)/(1 -s*q0)
Change in P = [pq(s2q -s1p)] / [1-(s1P^2) -(s2q^2)]
*if we reach equilibrium, change in p = 0
SO, pE = s2/(s1+s2) and qE = s1/(s2+s1)
3. Migration, Selection & Mutation
Saturday,*February*4,*2017 1:36*PM
Document Summary
Three factors that mess up hardy-weinberg equilibrium are migration, selection and mutation which alter allele frequencies. Movement of alleles from one population to the next. If allele frequencies are the same between two populations, migration has no effect. -> population 1 after migration: p1"= mp2 + (1-m)p1 = p1 + m(p2-p1) M is the migration rate = proportion of the total combined number of individuals in the new population 1 that came from population. Wild population of squirrels are all black [f(b)=1, f(b)=0]. A researcher releases grey squirrels [f(b)=0, f(b)=1] that make up 5% of the population. P1" = m(p2-p1) + p1 = 0. 05(0-1) + 1 = 0. 95. Q1= 1- p1" = 1- 0. 95 = 0. 05. Tracking proportion of individuals in combined population by origin: 1p1 = 0p1 (1-m) + mp2 kp1 = k-1p1 (1-m) + mp2. *m is assumed to be constant and the size of the native population is assumed constant as a proportion.