Engineering Science 1021A/B Lecture Notes - Lecture 32: Fastener, Viscoelasticity, Ductility
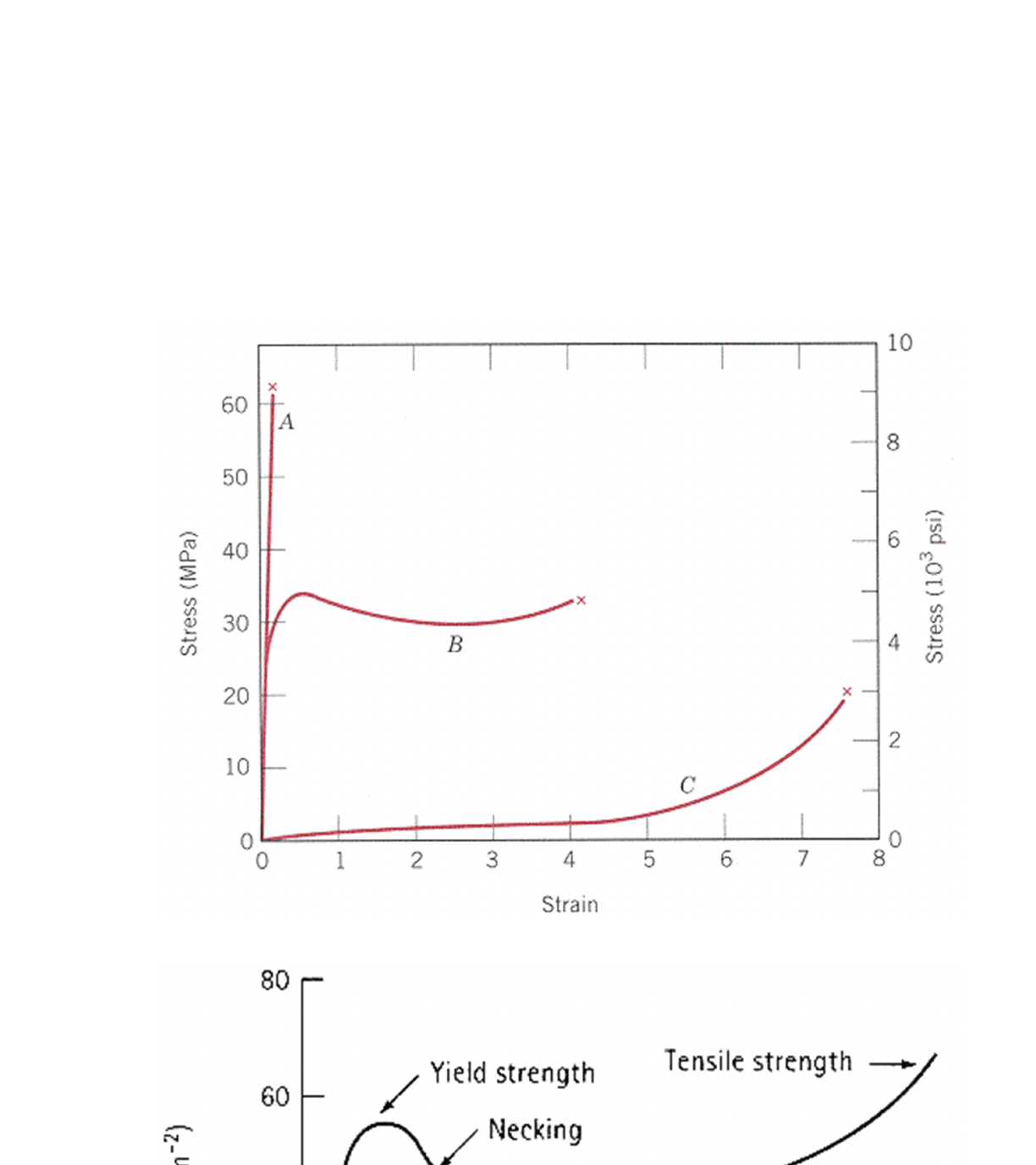
Polymers can be:
Elastic (Brittle
a.
Elastic (Plastic)
b.
Highly Elastic
c.
•
Deformation of Amorphous Polymers
•
Deformation of thermoplastics polymers is more complicated than in metals or
ceramics
It depends on the applied stress and the strain rate
○
•
Elastic deformation is the result of:
Stretching the covalent bonds of the chain
○
The rotation of the curved chains
○
•
On unloading, the strain due to:
Stretching the chains is recovered immediately
○
The rotation of the chains is recovered in a much longer time period
○
•
Plastic deformation in polymers does not occur by the motion of dislocations
Deformations is accomplished by the motion of polymer chains relative to
each other
If the polymer is not brittle, after yielding the chains begin to
disentangle, straighten and slide past each other
§
○
•
Necking begins almost immediately after yield, but is different than necking in
metals
•
Necking in Amorphous Thermoplastics
A neck occurs in amorphous thermoplastics as the polymer chains align•
Instead of being weaker because of the smaller cross-sectional area, the neck is
stronger because the chains are aligned and we are pulling on the now stronger
Van der Wall's bond and the covalent bonds of the backbone
•
Deformation of Semi-Crystalline Thermoplastics
Elongation occurs first in the amorphous regions between crystalline lamellae•
Crystalline lamellae begin to align in the direction of the applied stress and then
break into smaller segments
•
•
Under an applied stress, small voids can open up in bands perpendicular to the
applied load
Known as crazing
○
○
•
In transparent materials, these voids can appear as an opaque band through the
material
•
A craze is not a crack and can still support a stress•
As the voids elongate, the ligaments between them are subjected to higher
stress and eventually fracture causing the craze to grow
Eventually the crack grows to a size that causes rapid fracture
○
○
•
Time Dependent Deformation
Deformation is accomplished by the motion of polymer chains moving relative
to each other
•
Sliding polymer chains past each other take some time
If we apply the load slowly, the chains will slide easily
○
If the load is applied quickly, the chains do not have time to slide and the
polymer behaves in a brittle manner
○
•
This is viscoelastic behavior
@ high temperatures or low strain rates: more ductility
○
@ low temperature or high strain rates: less ductility
○
•
Creep and stress relaxation are time dependent phenomena•
Creep: change in length at a constant load•
Stress-relaxation: change in load at constant extension
Stress vs time @ a constant strain
○
•
•
•
The rate at which the stress decreases in the stress-relaxation process is given
by: ! " !#$ %&'()
*+
,: relaxation time (material property)
○
!#: initial stress
○
!: time dependent stress
○
•
Stress Relaxation Example
A stress of -./012 is applied to a polymer serving as a fastener in a complex
assembly. At a constant strain, the stress drops to -345/012 after 100 hours. If
the stress on the part must remain above 14.5 MPa in order for the part to
function properly, determine the life of the assembly.
! " !#$ %6'78
* 9
Find the relaxation time, ,
, " :-;;/<=>?@
ABC-345D:ABC-.D"EE5;/<=>?@
Time to reach ! " -F45/0G2
○
Temperature Dependence of Deformation
Increasing temperature:
Decreases young's modulus
○
Decreases the yield strength
○
Increases ductility
○
○
Due to Van der Waal's bonds are weak and east to melt
§
○
•
Relaxation Modulus
Viscoelastic Behavior for the load:•
The strain response can be:
○
This viscoelastic Relaxation Modulus is another way to characterize the stress
relaxation behaviour of a polymer
It is a time dependant Young's Modulus
○
•
Stress relaxation test measures stress vs. time at a constant strain•
Relaxation modulus is calculated as HICJD"KC7D
L
•
With time, the apparent stiffness of the material changes•
•
Deformation of Polymers
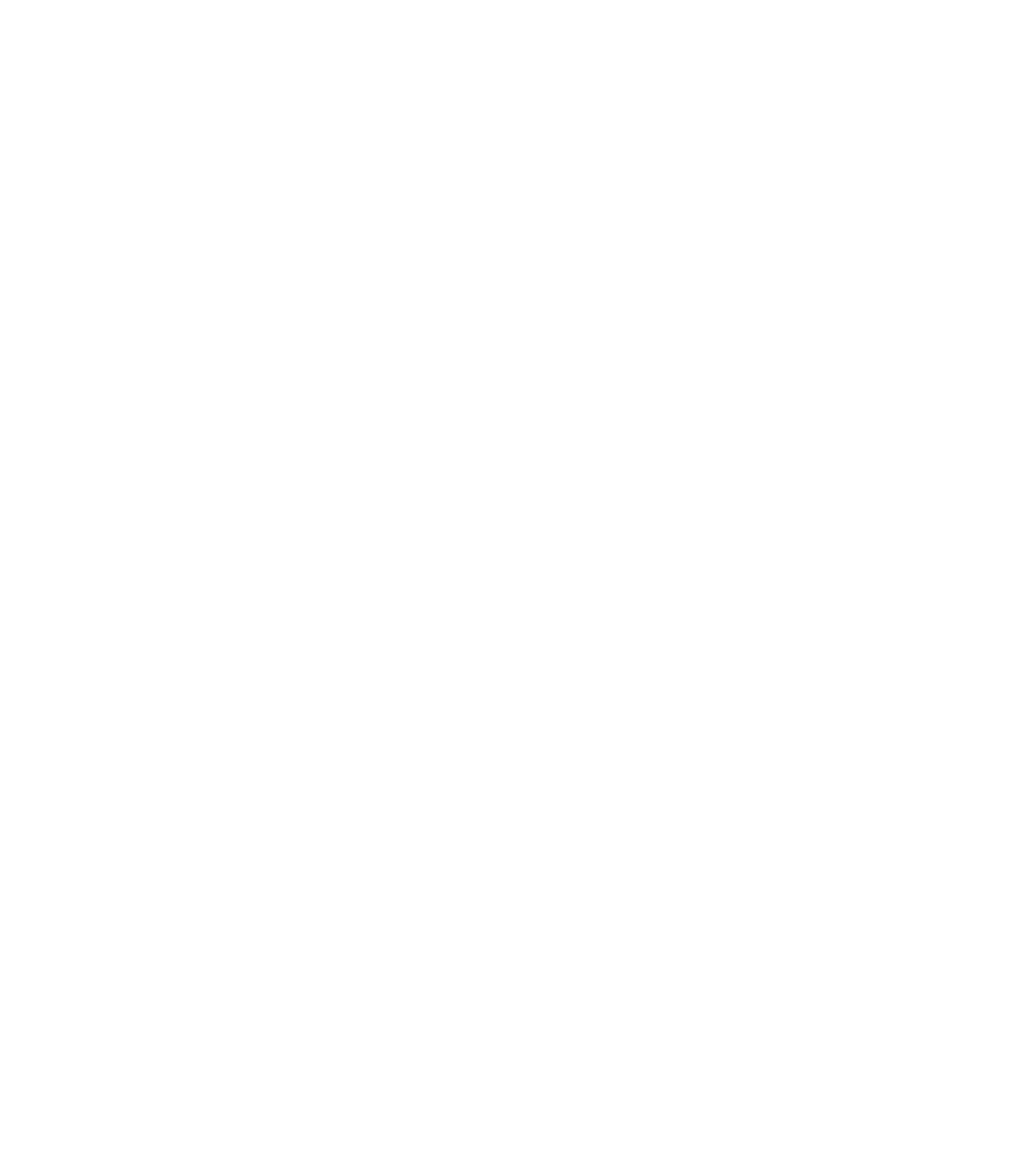
Polymers can be:
Elastic (Brittlea.
Elastic (Plastic)b.
Highly Elasticc.
•
Deformation of Amorphous Polymers
•
Deformation of thermoplastics polymers is more complicated than in metals or
ceramics
It depends on the applied stress and the strain rate
○
•
Elastic deformation is the result of:
Stretching the covalent bonds of the chain
○
The rotation of the curved chains
○
•
On unloading, the strain due to:
Stretching the chains is recovered immediately
○
The rotation of the chains is recovered in a much longer time period
○
•
Plastic deformation in polymers does not occur by the motion of dislocations
Deformations is accomplished by the motion of polymer chains relative to
each other
If the polymer is not brittle, after yielding the chains begin to
disentangle, straighten and slide past each other
§
○
•
Necking begins almost immediately after yield, but is different than necking in
metals
•
Necking in Amorphous Thermoplastics
A neck occurs in amorphous thermoplastics as the polymer chains align•
Instead of being weaker because of the smaller cross-sectional area, the neck is
stronger because the chains are aligned and we are pulling on the now stronger
Van der Wall's bond and the covalent bonds of the backbone
•
Deformation of Semi-Crystalline Thermoplastics
Elongation occurs first in the amorphous regions between crystalline lamellae•
Crystalline lamellae begin to align in the direction of the applied stress and then
break into smaller segments
•
•
Under an applied stress, small voids can open up in bands perpendicular to the
applied load
Known as crazing
○
○
•
In transparent materials, these voids can appear as an opaque band through the
material
•
A craze is not a crack and can still support a stress•
As the voids elongate, the ligaments between them are subjected to higher
stress and eventually fracture causing the craze to grow
Eventually the crack grows to a size that causes rapid fracture
○
○
•
Time Dependent Deformation
Deformation is accomplished by the motion of polymer chains moving relative
to each other
•
Sliding polymer chains past each other take some time
If we apply the load slowly, the chains will slide easily
○
If the load is applied quickly, the chains do not have time to slide and the
polymer behaves in a brittle manner
○
•
This is viscoelastic behavior
@ high temperatures or low strain rates: more ductility
○
@ low temperature or high strain rates: less ductility
○
•
Creep and stress relaxation are time dependent phenomena•
Creep: change in length at a constant load•
Stress-relaxation: change in load at constant extension
Stress vs time @ a constant strain
○
•
•
•
The rate at which the stress decreases in the stress-relaxation process is given
by: ! " !#$ %&'()
*+
,: relaxation time (material property)
○
!#: initial stress
○
!: time dependent stress
○
•
Stress Relaxation Example
A stress of -./012 is applied to a polymer serving as a fastener in a complex
assembly. At a constant strain, the stress drops to -345/012 after 100 hours. If
the stress on the part must remain above 14.5 MPa in order for the part to
function properly, determine the life of the assembly.
! " !#$ %6'78
* 9
Find the relaxation time, ,
, " :-;;/<=>?@
ABC-345D:ABC-.D"EE5;/<=>?@
Time to reach ! " -F45/0G2
○
Temperature Dependence of Deformation
Increasing temperature:
Decreases young's modulus
○
Decreases the yield strength
○
Increases ductility
○
○
Due to Van der Waal's bonds are weak and east to melt
§
○
•
Relaxation Modulus
Viscoelastic Behavior for the load:•
The strain response can be:
○
This viscoelastic Relaxation Modulus is another way to characterize the stress
relaxation behaviour of a polymer
It is a time dependant Young's Modulus
○
•
Stress relaxation test measures stress vs. time at a constant strain•
Relaxation modulus is calculated as HICJD"KC7D
L
•
With time, the apparent stiffness of the material changes•
•
Deformation of Polymers
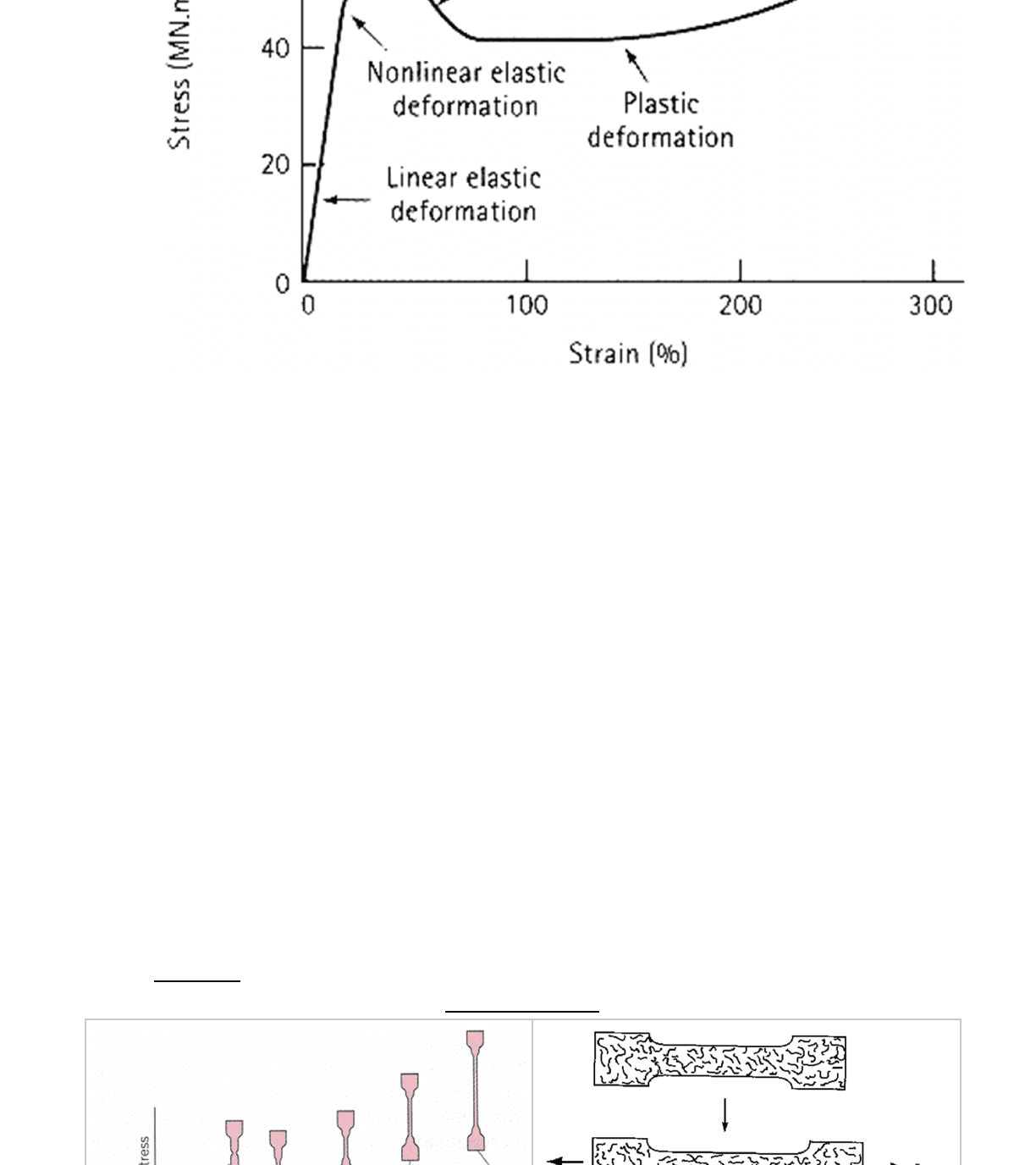
Polymers can be:
Elastic (Brittlea.
Elastic (Plastic)b.
Highly Elasticc.
•
Deformation of Amorphous Polymers
•
Deformation of thermoplastics polymers is more complicated than in metals or
ceramics
It depends on the applied stress and the strain rate
○
•
Elastic deformation is the result of:
Stretching the covalent bonds of the chain
○
The rotation of the curved chains
○
•
On unloading, the strain due to:
Stretching the chains is recovered immediately
○
The rotation of the chains is recovered in a much longer time period
○
•
Plastic deformation in polymers does not occur by the motion of dislocations
Deformations is accomplished by the motion of polymer chains relative to
each other
If the polymer is not brittle, after yielding the chains begin to
disentangle, straighten and slide past each other
§
○
•
Necking begins almost immediately after yield, but is different than necking in
metals
•
Necking in Amorphous Thermoplastics
A neck occurs in amorphous thermoplastics as the polymer chains align
•
Instead of being weaker because of the smaller cross-sectional area, the neck is
stronger because the chains are aligned and we are pulling on the now stronger
Van der Wall's bond and the covalent bonds of the backbone
•
Deformation of Semi-Crystalline Thermoplastics
Elongation occurs first in the amorphous regions between crystalline lamellae•
Crystalline lamellae begin to align in the direction of the applied stress and then
break into smaller segments
•
•
Under an applied stress, small voids can open up in bands perpendicular to the
applied load
Known as crazing
○
○
•
In transparent materials, these voids can appear as an opaque band through the
material
•
A craze is not a crack and can still support a stress•
As the voids elongate, the ligaments between them are subjected to higher
stress and eventually fracture causing the craze to grow
Eventually the crack grows to a size that causes rapid fracture
○
○
•
Time Dependent Deformation
Deformation is accomplished by the motion of polymer chains moving relative
to each other
•
Sliding polymer chains past each other take some time
If we apply the load slowly, the chains will slide easily
○
If the load is applied quickly, the chains do not have time to slide and the
polymer behaves in a brittle manner
○
•
This is viscoelastic behavior
@ high temperatures or low strain rates: more ductility
○
@ low temperature or high strain rates: less ductility
○
•
Creep and stress relaxation are time dependent phenomena•
Creep: change in length at a constant load•
Stress-relaxation: change in load at constant extension
Stress vs time @ a constant strain
○
•
•
•
The rate at which the stress decreases in the stress-relaxation process is given
by: ! " !#$ %&'()
*+
,: relaxation time (material property)
○
!#: initial stress
○
!: time dependent stress
○
•
Stress Relaxation Example
A stress of -./012 is applied to a polymer serving as a fastener in a complex
assembly. At a constant strain, the stress drops to -345/012 after 100 hours. If
the stress on the part must remain above 14.5 MPa in order for the part to
function properly, determine the life of the assembly.
! " !#$ %6'78
* 9
Find the relaxation time, ,
, " :-;;/<=>?@
ABC-345D:ABC-.D"EE5;/<=>?@
Time to reach ! " -F45/0G2
○
Temperature Dependence of Deformation
Increasing temperature:
Decreases young's modulus
○
Decreases the yield strength
○
Increases ductility
○
○
Due to Van der Waal's bonds are weak and east to melt
§
○
•
Relaxation Modulus
Viscoelastic Behavior for the load:•
The strain response can be:
○
This viscoelastic Relaxation Modulus is another way to characterize the stress
relaxation behaviour of a polymer
It is a time dependant Young's Modulus
○
•
Stress relaxation test measures stress vs. time at a constant strain•
Relaxation modulus is calculated as HICJD"KC7D
L
•
With time, the apparent stiffness of the material changes•
•
Deformation of Polymers
Document Summary
Deformation of thermoplastics polymers is more complicated than in metals or ceramics. It depends on the applied stress and the strain rate. The rotation of the chains is recovered in a much longer time period. Plastic deformation in polymers does not occur by the motion of dislocations. Deformations is accomplished by the motion of polymer chains relative to each other. If the polymer is not brittle, after yielding the chains begin to disentangle, straighten and slide past each other. Necking begins almost immediately after yield, but is different than necking in metals. A neck occurs in amorphous thermoplastics as the polymer chains align. Instead of being weaker because of the smaller cross-sectional area, the neck is stronger because the chains are aligned and we are pulling on the now stronger. Van der wall"s bond and the covalent bonds of the backbone. Elongation occurs first in the amorphous regions between crystalline lamellae.